Dear Dr. Zoomie – I liked the piece you wrote about how particle accelerators work; I was wondering if all accelerators work the same way or if there’s other ways to make them.
Accelerator-on-a-chip
Would you believe I was just reading about a particle accelerator that will fit on a penny? It doesn’t reach very high energies, but it can boost electrons to energies of a few to several tens of thousands of electron volts (keV). That’s not a very impressive energy from the standpoint of, say, the Large Hadron Collider at CERN, which accelerates larger particles to energies about a billion times higher. Compared to that, a paltry few tens of electron volts don’t seem like much, but when we consider that the LHC is 27 km (27 million mm) in circumference while the micro-accelerator is only a half mm long, that energy looks much more impressive – especially when we consider that it uses a novel method for accelerating electrons.
Most accelerators use pulsing magnetic fields created by powerful superconducting magnets cooled by liquid helium to temperatures very near to absolute zero. Instead, the tiny nanophotonic accelerator shines miniature lasers at near-microscopic pillars lining a vacuum tube only a hundredth the width of a human hair. When the lasers interact with the pillars they produce an electrical field that accelerates the electrons to those energies in a fraction of an inch.
But (I hear you wondering) what good are electrons that are only a paltry few tens of keV? Why even bother?
Well…let’s start with the fact that this is the first generation of a brand-new technology, and that few tens of keV is only going to improve with time. Researchers are already suggesting schemes to use different materials, or chaining the micro-accelerators together to reach higher energies. This is nice, but these are still unlikely to ever produce more than a fraction the energy levels of the full-sized accelerators. Having said that, even a tens of keV is useful. A decade or so ago, when I was working in medical radiation safety, I observed dozens of procedures involving the implantation of small radioactive “seeds” into prostate tumors. The nuclide used, I-125, emitted radiation of only a few tens of keV – low, but enough to destroy the cancerous tissues. These accelerators, producing the same energies, can do the same, raising the possibility that – one day – radiation therapy might be administered through a catheter rather than a high-dose rate beam or implanted radioactive sources.
Cosmic shocks
And then we can go to the other extreme – from a tiny device that can fit inside a catheter to massive shock waves that accelerate charged particles to – literally – astronomically high energies. Recent observations have started to answer questions that have bothered cosmic ray astronomers for a few decades – wondering what mechanism can accelerate particles to within a whisker of the speed of light, to energies so high that a single atom carries as much energy as a quickly thrown pebble. That doesn’t sound very impressive – but, remember, we’re talking about a single atom that carries as much energy as an object containing a billion, trillion atoms. That’s impressive – and it’s even more impressive that we’re finally elucidating the mechanisms that make it possible.
One mechanism, Fermi acceleration (also known as Diffusive Shock Acceleration), was figured out several decades ago; shock waves moving through space often contain magnetic fields; when a charged particle is swept up by one of these shock waves the magnetic field can push the particle back out again at a higher energy. The particle can continue picking up energy this way if it encounters another shock wave – under the right circumstances the particle can bounce back and forth between shock waves, picking up more energy with each encounter. The problem is that nobody knew how the particles were injected into the shocks – only particles that have substantially more energy than the average particles in that part of space are able to cross the shock at all and, unless the particle can cross the shock it can’t take advantage of the acceleration mechanism. The “injection problem” (as this dilemma is termed) calls for a different acceleration mechanism – the one that scientists just figured out.
This one is referred to as a reinforced acceleration mechanism, and it also includes plasma, magnetic fields, as well as some electromagnetic “surfing” in which charged particles can pick up energy by riding magnetic fields propagating through electrically charged gas.
What’s interesting is the comparison to high-energy accelerators we construct here on Earth. Most accelerators use several stages to boost particles from rest to high energies. For example, a van de Graaf accelerator might increase particle energies to the point that they can be injected into a cyclotron; the cyclotron boosts the energy to the point where they can be injected into the main accelerator ring. Similarly, with the highest-energy cosmic rays, it seems as though one mechanism increases particle energies to the point at which they can take advantage of different mechanisms that will accelerate them even more. And every step of the way the acceleration takes place without the particles colliding with other particles – they simply ride the electromagnetic waves all the way up to from garden-variety energies to truly extraordinary peaks.
Laser-plasma (“wakefield”) accelerators
The accelerator-on-a-chip is sort of cute and the multi-stage cosmic accelerators are simply awe-inspiring; the laser-plasma accelerator falls somewhere in between.
A plasma is an electrically charged gas, a fluid of negatively charged electrons and positively charged ions; the electrical field of a plasma can be used to accelerate charged particles to high energies in a short distance; a laser at the University of Texas at Austin reached an energy of 2 GeV (two billion electron volts) in a distance of just under an inch and a design at the Lawrence Berkeley Laboratory reached twice that energy.
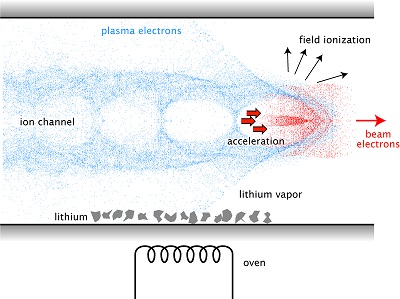
Plasmas are normally a relatively uniform mixture of electrons and ions, but they can be manipulated to separate the light electrons from the heavier ions. This establishes an electrical field gradient that can be used to accelerate charged particles. Shooting a beam of electrons or protons through the plasma creates an electromagnetic “wake” that accelerates the charged particles to even higher energies. This same phenomenon can be induced by a laser pulse passing through the plasma.
If the electrical field of the electrons or protons, or the laser pulse, is strong enough it can expel the electrons to form a charged “bubble” that moves through the plasma at nearly the speed of light, pulling a cluster of particles in its wake. And if the particles that are to be accelerated are injected at an angle across the “face” of the wake, they can “surf” the electromagnetic wave to accelerate to even higher velocities.
Wakefield accelerators show tremendous potential – but the technology is still fairly new and physicists and engineers are still working out the bugs. If they can do so, these could very well be the next generation of powerful accelerators, reaching high energies, possibly in a device that could sit on a desktop.
Image References
- Illustration Plasma Wakefield Acceleration:
https://en.wikipedia.org/wiki/User:Rasmus_Ischebeck
https://commons.wikimedia.org/wiki/File:Illustration_Plasma_Wakefield_Acceleration.png