Hi, Dr. Zoomie – I saw a couple stories about NASA building nuclear rockets and maybe sending nuclear reactors to the Moon. Is it safe to have nuclear reactors so close to so many people? And what if there’s an accident at launch or the reactor burns up in the atmosphere? Should I be worried?
Great question! Believe it or not, there have been nuclear reactors shot into space for decades, and one even burned up on reentry (as have some radioactive sources)…all without hurting or killing anybody. Not only that, but we’ve had people working in close proximity to hundreds of nuclear reactors for nearly 70 years; while there have been some serious accidents on some Soviet/Russian submarines, no other navy has reported (or been found to have had) a nuclear reactor accident that harmed any sailors.
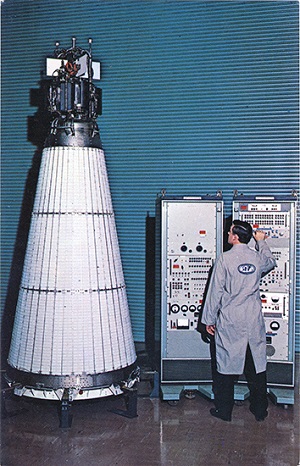
This is the short answer – let’s dig down a little to learn a bit more. And for a short primer on how nuclear reactors work, you can check out something I wrote in July (https://www.ntanet.net/the-reactor-zoo-part-1-the-different-types-of-nuclear-reactors/)
Let’s start with why NASA is looking at using nuclear-powered spaceships and reactors on the Moon.
A lot of what it comes down to is energy density – how much energy you can provide per cubic centimeter (or per kilogram) of fuel and engine. Gasoline, for example, produces about 35 million joules (MJ) of energy per liter of gas, or a bit more than 50 MJ per kg of gasoline. In more familiar units, 50 MJ is about 14 kilowatt-hours (kWh) or close to 50,000 BTU – and this does not include the weight of the engine or tanks needed to burn the gasoline. The most efficient chemical fuel by weight is hydrogen, which produces over 140,000 MJ per kg – since hydrogen is so light, though, even liquid hydrogen (the densest form) only produces 10 mJ for each liter burned. By comparison, fission of weapons-grade uranium produces about 79 million MJ/kg – about 6000 times more than liquid hydrogen https://www.whatisnuclear.com/energy-density.html). So even though the reactor itself is heavier than a combustion chamber, the incredibly high power density makes uranium fission an attractive way to power a lunar colony or to propel a rocket to Mars – especially when you remember that the fuel doesn’t need to be replaced very often.
Having said that, a nuclear rocket still needs to shoot something out the back in order to move (that’s Newton’s Third Law of motion – https://www1.grc.nasa.gov/beginners-guide-to-aeronautics/newtons-laws-of-motion/) – for a nuclear rocket, what’s shot out the back is some fluid (liquid or gas) that’s heated to high temperatures by the nuclear reactor; when heated, liquids will vaporize and increase their volume by a few orders of magnitude, while hot gases also increase their volume substantially – either way, the hot gas will blast out the back, shoving the rocket forward. So a nuclear rocket still needs to carry fluid around with it – but the engineers can choose a fluid that’s light, that absorbs heat readily, and that expands greatly – it need not also be flammable. Nuclear reactors need a fluid of some sort too, to keep the core cooled and to transfer the energy of fission to something that will produce energy (such as boiling water to form steam to turn a power turbine), but this fluid circulates in a closed loop and does not need to be replenished as often.
I should also mention that, while we’re all familiar with spacecraft sprouting “wings” of solar panels, solar power is only useful in the inner solar system – out to about Mars – and that it needs vast expanses of solar cells to produce enough power to run the ISS; long-haul space missions will require more power than the ISS, and when we add the need to propel the ship out of earth orbit, to brake into Mars orbit, and the reverse on the way home, solar cells just won’t do the trick. And if we want to explore the outer solar system, especially when we start sending people out that way, nuclear reactors give us a lot more power and the ability to maneuver around the moons and rings of a planetary system more effectively than can today’s spacecraft.
So this is the “why” part of nuclear reactors in space. Now let’s talk about safety, beginning with safety to use here on Earth.
Safety to those on Earth
Let’s start with launch-related and reentry-related concerns. And, believe it or not, this issue has not only been considered and designed for, but it’s also been “tested” to some extent. With regards to the design – the US designed space-going nuclear reactors in the 1960s, as did the Soviet Union. The American designs included NERVA (Nuclear Energy for Rocket Vehicle Applications) in the 1960s, Rover in the 1950s and 1960s, Project Timberwind in the late 1980s, and Prometheus in the early 2000s. Although none of these went into space, another American reactor, the SNAP (Systems for Auxiliary Nuclear Power) did make it into orbit; SNAP 10A was launched in 1965, operating successfully for 43 days before an electrical component (non-nuclear) malfunctioned; the reactor remains in orbit about 700 nautical miles high. This was the only reactor to date launched into space by the US.
Somewhat more interesting was the Soviet nuclear reactor program, which launched over 30 low-energy fission reactors into space in the 1960s and 1970s and flew the next-generation TOPAZ reactor in 1987. Yet another Soviet-designed reactor was loaded onboard a RORSAT maritime reconnaissance satellite that was launched in 1977 and came down in Canada in 1978, scattering contamination over a wide area in the Northwest Territories near the town of Fort Resolution. Cleanup of this took several years and the Canadian government sent the Soviet Union a cleanup bill of CAN $6 million (the USSR gave themselves a 50% discount, paying just $3 million Canadian dollars).
Finally, the US had one satellite that, while not powered by a nuclear reactor, was powered by large amounts of plutonium (Pu-238), the heat of which was used to provide energy for the craft. SNAP 9A was launched in April 1964 and reentered the Earth’s atmosphere when it failed to achieve orbit. SNAP 9A burned up during reentry, scattering much of its Pu-238 over the Indian Ocean. While most of the plutonium drifted down over the ocean, some was widely distributed across the Earth (https://www.nature.com/articles/srep15707)…with no apparent health effects on populations anywhere. In addition to SNAP 9A, a similar source on the Apollo 13 Lunar Module reentered the Earth’s atmosphere when the LM made an unplanned return to Earth in its role as the crew’s lifeboat when the craft suffered an explosion on its way to the Moon. In this case, though, the source capsule remained intact and it current resides (safely!) at the bottom of the South Pacific Ocean in the vicinity of the Tonga Trench.
The thing is, launchpad explosions and unanticipated reentries are not novel ideas – we’ve seen enough rockets and missiles blow up (some with tragic loss of life) and enough satellites unexpectedly reenter the Earth’s atmosphere that making allowances for such events is sort of a no-brainer. And even though these events are hot and destructive, radioactive sources and nuclear reactors can be designed to withstand most of these stresses to safeguard the radioactive and nuclear materials inside. In addition, the launch trajectories and orbits can be planned to avoid most landmasses and, often, even an unplanned reentry can be planned to come down in an area that will cause the least risk to those on the ground. The fact is that, of the multitude of launches involving radioactive or nuclear materials (and the few failures), there’s no indication that anyone on Earth has been harmed. So I think it’s safe to feel that the launch of a nuclear rocket to Mars or of nuclear reactors to the Moon will continue this trend.
The Crew
Safety for astronauts riding on a nuclear-powered rocket is a reasonable concern. But I’d suggest that this is a problem that was solved decades ago – American (and British, French, Chinese, and Russian) sailors have been riding in close proximity to operating nuclear reactors since the mid-1950s and, with the exception of some Russian reactor accidents, apparently without significant health effects, especially in the American, British, and French submarines (there’s not as much information released about the Russian and Chinese navies) https://journals.lww.com/joem/Fulltext/2022/02000/Mortality_of_Enlisted_Men_Who_Served_on.6.aspx. What this means is that we know how to make reactors that are safe to work next to for long periods of time at sea. And, while there is, of course, a difference between the environment in space versus at sea, this knowledge can transfer from one environment to the other. For reactors powering lunar stations the safety is even greater because the reactors can be placed at a distance from the base to reduce radiation exposure even further. Oh – and with regards to unexpected equipment failures…while they do happen, the commercial nuclear reactors have an availability of greater than 90% (meaning that they are online and operating over 90% of the time), and military reactors are even more reliable.
Putting it all together
So – first – you’re right to be concerned; that’s demonstrated by the fact that NASA was concerned enough to study and design for these issues, the fact that we’ve seen spacecraft and rockets explode on launch, that we’ve seen spacecraft (including nuclear-powered craft) reenter unexpectedly, and because the Navy was concerned enough to spend a lot of time studying the safety of submarine reactors. This all means that your concerns are not only well-founded, but that they’ve been shared by a lot of people who design and build these things for a living. And our experience seems to show that, while our concerns are well-founded, we don’t necessarily need to be worried.