Dr. Zoomie! Going through the cabinets of a few recently retired engineers at work I found some things that look to be old instruments – maybe from the 1930s or 1940s? I know that a lot of instruments in those days used radium paints to make them easier to read in the dark – is that something I need to worry about here? And if they are painted with radium paint, what sort of radiological could there be?
Very nice! The instrument in the image you’ve sent is a Keuffel & Esser “Aero-1938-USN” bubble-sextant head that the Navy fixed into a pilot-balloon (PIBAL) theodolite cradle. The same optical head was hand-held for air navigation, but in this deck-mounted version it let aerology crews track weather balloons. In its original hand-held form the bubble-sextant head was critical for celestial navigation aloft; mounted in the PIBAL cradle it served a different mission—measuring balloon angles once a minute to compute winds aloft.
Bubble-sextant designs like this one were even taken into space during the Apollo program as a backup in case the Soviets disrupted communications between Apollo and Mission Control. This particular model, the Aero-1938, was built by Keuffel & Esser Co. of New York, likely around 1945, and features both radium-based luminous paint and thorium-doped optics.
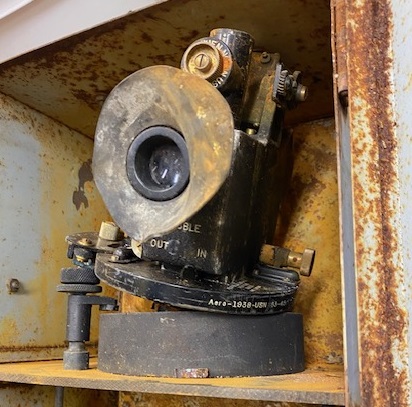
Bubble sextant heads like this were known as “bubble sextants” because, to keep them absolutely level when making readings, the operator centered a small bubble on a glass dome built into the instrument. Sextants are used to measure the angle between the horizon and various celestial objects; the only way to ensure that these angles are being measured accurately is for the sextant to be level. And, because readings were taken at all hours of the day and night, glow-in-the-dark paint was applied to the scales and numbers the operator needed to read and record. This paint used radium for many years, until it was phased out and replaced with tritium.
So that’s the instrument – let’s talk about its objective lens
The sky-facing objective lens—part of the sextant’s optical system—leads into another fascinating use of radioactive material: thorium-doped glass. Just as lead can be added to glass to make it beautiful (e.g. lead crystal) by changing its optical qualities, adding thorium to glass also changes the optical properties such that thoriated lenses – containing between about 15-30% thorium (lead crystal contains a comparable amount of lead) – make for superior optics.
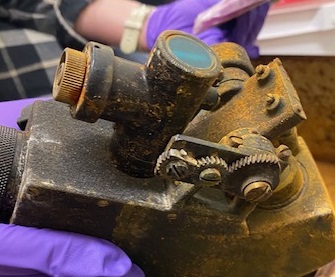
Thoriated lenses were invented in the 1930s and quickly found their way into every kind of precision military optic—bomb-sights, periscopes, reconnaissance cameras, and, after the war, the ultra-fast still and cine camera lenses that photographers came to covet. While the photos were great, though, one had to be careful not to leave the lens on a loaded camera for prolonged periods of time to minimize fogging of the photographic film by radiation from the thorium. Not only that, but the thorium could cause the glass itself to turn brown over time due to radiation damage – interestingly, I’ve seen the same thing through a petrographic (rock and mineral) microscope. What happens is that small mineral grains called zircons are frequently embedded in larger grains of biotite or muscovite (forms of mica); zircons have a geochemistry that includes traces of uranium and thorium and the radiation from these elements damages the crystal, causing the brown color. This same process is acting on the glass of the lens.
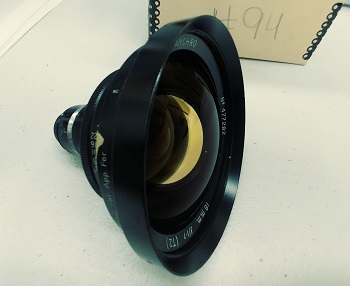
From a radiation-safety standpoint, the only real concern with thorium-glass optics is a camera with an eyepiece using thoriated glass – these could give a high radiation exposure to the lens of the eye (as high as a few tens of rads to the cornea) from the alpha radiation, although dose to the lens of the eye would be much lower because alphas are so weakly penetrating in tissue. The photographer would also receive some exposure simply from carrying the camera, but much less than any exposure that could cause health issues. And from a regulatory standpoint, thoriated camera lenses are exempt from regulation.
Turning back to the radium-painted sextants and the radiation-safety concerns they raise, in an earlier article on the Golden Age of Radium, I described surveying a U.S. Navy Aircraft Sextant, Mark V (Pioneer Instruments/Bendix). This instrument gave readings of up to 3 mR/hr on contact with the device, but nothing above background at a distance of one meter. I’m not sure if these are typical readings or not—I just don’t have a large set of data for these things. But if this is a typical reading, then these sextants, and other instruments with similar or lower radiation levels, don’t emit dangerous levels of radiation. Here’s the thing, though—radium is fairly radiotoxic if it’s inhaled or ingested, and radium paint is known to start to flake off over the decades. If the glass cracks that’s covering any of the luminous dials, radium-bearing dust can escape, spreading trace amounts of contamination. In fact, this was a minor concern on nuclear submarines since our divers were issued radium-dial watches; if any of the divers’ watches suffered a cracked or broken dial we needed to check for contamination to find out if it was leaking radioactivity. Luckily I never had to deal with that during my time onboard!
Regulation of radium-painted instruments is sort-of nebulous. Radium-226 occurs in nature and the states (not the NRC) regulate Naturally Occurring Radioactive Materials (NORM). Except that Ra-226 is categorized as “byproduct material,” which is regulated by the NRC. In the federal regulations, 10 CFR 30.15 gives exemption limits for radium, but it only mentions Ra-226 in timepieces (1 microcurie per timepiece in intact timepieces manufactured prior to November 30, 2007), but not in aircraft or other instruments. I have to admit I didn’t have a chance to do an exhaustive search of the regs so I can’t give a definitive answer. But by and large, you shouldn’t need to worry about the regulators breaking down your door (or sending you a sharply worded letter, which is less unlikely) over an instrument with a radium-painted dial, as long as it’s not leaking.
So – with your instruments – check them for leakage from time to time and definitely don’t lick the dials! If you have a lot of them, consider putting them in a cabinet or on a shelf that’s a few meters from where people spend a lot of time sitting or sleeping. But aside from that, just enjoy them.