On the other end of the electromagnetic spectrum from x-ray and gamma radiation lie radio waves. And even though radiofrequency radiation isn’t ionizing, it can be dangerous – in the Navy I heard many stories of seagulls flying through a radar beam and falling from the sky, or of sailors being injured by high-energy radar systems. And outside of the military it’s not unusual for a radiation safety officer to find him- or herself responsible for the safety of those working with or around microwave and radio emitters. Don’t worry – this post isn’t about how to keep people safe from radar sets, radio transmitters, or the like (although I should note that a strong radar set can injure of kill a person who stumbles into the beam and that it’s not uncommon for electromagnetic interference to produce alarmingly high radiation readings) – what I’m going to talk about, in keeping with the recent theme, is some of the neat work radio astronomers are doing and how they’re using radiofrequency radiation to tease out the life history of quasi-stellar objects (QSOs) that emit various levels of radiofrequency radiation. And perhaps a good place to start is with what a QSO is.
Spiral galaxies look like a pinwheel with lovely spiral arms arching out from a central ball of stars; at the very center of this ball often lies a mindbogglingly massive black hole that weighs anywhere from hundreds of thousands to billions of times as much as our Sun. The gravity from so massive an object acts almost like a cosmic vacuum cleaner, sucking in gas, planets, and even stars from light years away; like water swirling down a drain, all of this material swirls around the black hole, gradually working its way from the periphery to the event horizon, into which it vanishes, never to be heard from again.
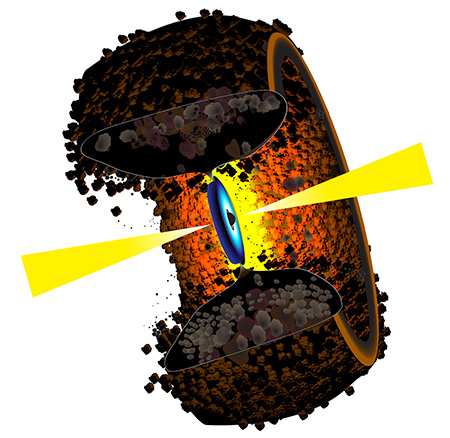
As this gas accumulates it heats up and the heat can cause some of the gas to be blasted out into space. If conditions are right, a spinning black hole will have a strong magnetic field that confines this gas into two narrow beams shooting out into space in opposite directions. The regions in which this happens (the black hole’s accretion disc and the source of the jets in those galaxies that develop them) are tremendously bright, looking starlike in most telescopes. They are so bright, in fact, that the first astronomers to see them failed to see the surrounding galaxy, just as we have difficulty seeing an automobile when the headlights are shining in our eyes. Since they looked star-like, these were named “quasi-stellar objects” – QSOs.
The gas swirling around a black hole is tremendously hot – millions of degrees; so hot that it gives off x-rays. As the gas expands into the space between galaxies it cools off; at the same time, it is also laced with magnetic fields. This combination can cause it to emit radio waves (radiofrequency radiation). In fact, these radio waves give us one of the primary distinguishing features of QSOs – they are often divided into “radio-loud” (RL) and “radio-quiet” (RQ) objects. And this takes us to Marina Arnaudova, a post-doctoral fellow at the Centre for Astrophysics Research, University of Hertfordshire in the United Kingdom. Marina and her colleagues are studying QSOs using the radio waves they emit to try to learn more about their earlier years, including identifying some of the factors that drive them to be radio-quiet or radio-loud.
Working with huge amounts of data, Marina and her colleagues were able to “stack” the spectra from a large number of QSOs of varying degrees of radio “volume.” After correcting them for many factors, including the rate at which the objects are receding from us (the universe as a whole is expanding and more-distant objects are retreating more quickly than those that are nearer) – what they found is that there is not really a clear-cut distinction between objects that are loud or quiet; rather, they seem to lie on a continuum. They were able to rule out some possible explanations (e.g. the mass of a galaxy’s central black hole or the rate at which gas is being added to the swirling disc surrounding it), leaving fewer explanations to consider. They also noticed that many of the RL objects seem to reside in galaxies that are forming stars at a higher rate than their quieter cousins. Ultimately, they suggest two possible explanations: that a rapidly spinning black hole might be powering the radio emissions of the RL objects, or that the large amounts of gas and dust in younger galaxies is simply blocking our view – revealing the area in which these jets originate as the galaxy ages and the dust and gas are cleared out. To figure out which explanation is correct will require some more study.
And finally, I feel obligated to add a few comments of my own. First is that, as adventurous as I feel myself to be, I’m not sure I’d want to be anywhere close to the areas that Marina and her colleagues are studying. The x-ray radiation dose rates from the hot gas would be high enough to fry any of us fairly quickly and, further out, I’m pretty sure that radio emissions that are strong enough to be seen across billions of light years would do a good job of curdling my brains. I’m happy to leave these phenomena at much greater than arm’s length!