Good morning Dr. Z! So I’m curious…I’m visiting a buddy in Richland Washington and he took me to see something called the “B Reactor” from the Manhattan Project. I guess that’s where they made the plutonium that was used in one of the atomic bombs dropped in WWII. It was pretty cool – but I’m not quite sure why it’s such a big deal that they made it into a historical monument. Even after reading about it on Wikipedia I still don’t quite get it – can you help me out? Thanks!
Wow – I’m envious! I’ve visited the B Reactor (https://www.nps.gov/thingstodo/hanford-attend-a-b-reactor-tour.htm) myself and would love to spend more time there. Not only is it a piece of history, but it also reflects a huge (and hugely expensive) gamble at a time when world history might well have turned on whether or not a scientific hunch could be engineered into being, and whether or not it would work. Here’s why…and why it’s such an important site.
We’re going to start with uranium – the first fuel that was considered for making nuclear weapons. As I’ve mentioned in some earlier postings, uranium comes in two “flavors” – U-238 makes up 99.2% of all the world’s natural uranium and most of the rest (about 0.72%) is comprised of U-235. This fact, as stated, earns a well-deserved “So what?” The thing is, it’s the rare atoms of U-235 that fission, which means that, to make a nuclear weapon, the small number of U-235 atoms have got to be plucked out of the large mass of U-238; to put it more technically, the U-235 has got to be enriched to the point at which it can be made into a weapon. Realizing this was easy – implementing it was fiendishly difficult.
Here’s the thing…. Think about mining – the miners dig up a bunch of ore that contains, say, tin. The tin will be part of a mineral and, in order for it to be useful, it’s got to be removed from the ore. But the tin will be present as part of a molecule – the tin mineral cassiterite is composed of tin and oxygen (specifically, it’s SnO2). For the tin to be useful, the cassiterite has to be chemically processed to remove the oxygen atoms so that we have pure tin. That, in turn, can be used – it can be added to copper to form bronze, it can be made into tin foil (or other products using metallic tin), or it can be added to other chemicals to form other compounds for other purposes. The reason we can do this is because atoms of tin act differently – they have different chemical properties – than do atoms of oxygen (or any other element). Isolating the tin atoms – enriching the concentration of tin – might be challenging, but it’s a relatively straight-forward thermal and chemical process. But, again, U-235 is chemically identical to U-238, which means that we can’t use chemistry to get a “handle” on the U-235 atoms – enriching uranium is harder than enriching tin.
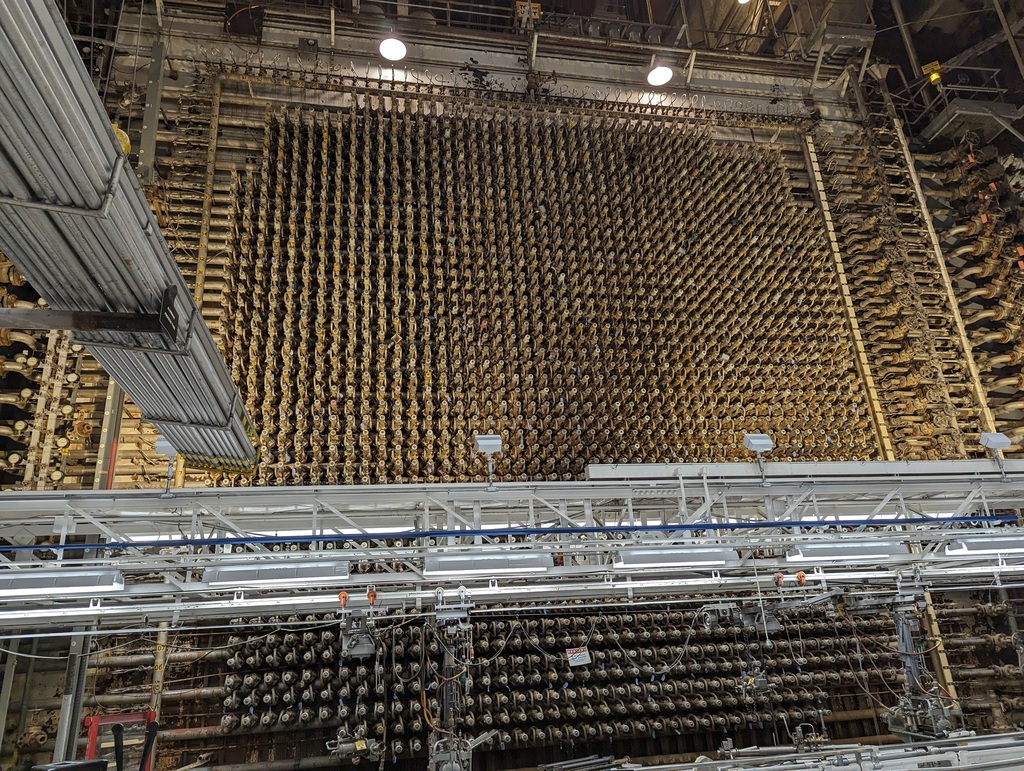
Inside a vast chamber, an awe-inspiring 11-meter high grid of over 2,000 cylinders stands tall; this is the heart of a nuclear reactor. Here, the plutonium was created that fueled the “Fat Man” atomic bomb, which decimated Nagasaki, Japan, in 1945.
In fact, uranium enrichment has got to be done physically – taking advantage of the sole difference between these two isotopes…their respective weights. And since that difference in weight is so very slight, enriching U-235 to the high concentration required to make a nuclear weapon requires processes involving thousands or tens of thousands of steps – it used to be gaseous diffusion, but today it’s more likely to be done using tens of thousands of centrifuges, each one producing the tiniest incremental degree of enrichment before passing the uranium on to the next step.
Now – put yourself in the shoes of the folks managing the Manhattan project. They know that uranium will sustain a chain reaction – but, even though they’re pursuing multiple avenues for enriching uranium to weapons grade, most of these techniques are pushing the envelope with regards to the state of the art for engineering, process control, and physics and there’s no guarantee that any of these methods will come to fruition in time to aid the war effort. Luckily, in their experiments, the scientists have observed that bombarding uranium with neutrons in a nuclear reactor sometimes leads to the capture of a neutron (neutrons are emitted by fissioning uranium atoms) by a U-238 atom to form U-239 which, in turn, undergoes two radioactive decays to form an atom of plutonium – Pu-239 to be precise. Not only that, but Pu-239 fissions just as eagerly as does U-235…and plutonium just might be different enough from uranium to let us use chemistry to pull it out of the irradiated fuel. So the folks managing the Manhattan Project decided to try a second path to nuclear weapons – designing a nuclear reactor to produce plutonium and inventing the chemical process necessary to separate the plutonium from the rest of the fuel.
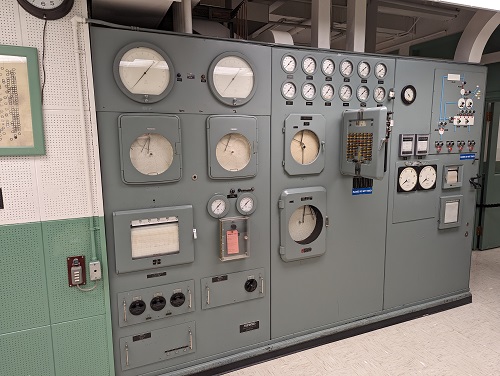
And now – take a minute to think about this. There is plenty of uranium on Earth and we already had a reasonably good understanding of how to work with it. But separating out the useful part was so difficult that the scientists and engineers thought that it might actually be easier to design the world’s first large nuclear reactor and to use it to produce a brand-new element about which we knew almost nothing – so if this new element could be produced, it would still be useless unless we could learn about its chemistry and then invent a way to process the highly radioactive reactor fuel to remove the plutonium, and then to further process it to turn it into a metal and to learn how to work the metal – they were proposing to invent a new type of reactor as well as inventing a new chemical engineering technique and new metallurgy to process a new chemical element. Just typing this, I’m shaking my head, marveling at the audacity of the project as well as gaining a new appreciation for the difficulty of uranium enrichment that such an undertaking would seem reasonable.
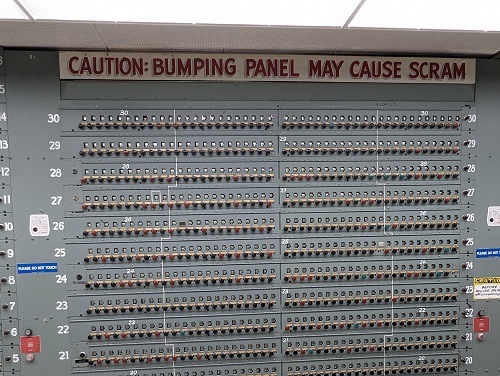
Skipping ahead, the B reactor was designed from the start to produce plutonium-239; like Fermi’s earlier reactor, it used graphite to slow the neutrons down to the point where they could be captured by the uranium atoms, although this reactor was large enough (250 MW) that it required water cooling to keep it from melting down. The slugs of uranium were pushed into the front of the core where the U-235 atoms fissioned and some of the neutrons from fission went on to form the Pu-239; after the uranium slugs had been in the core long enough to produce a useful amount of plutonium (a few grams or so), they were pushed out the back and into a 20-foot deep trough filled with water – the water absorbed enough radiation to keep the operators safe. After a few months, the slugs had undergone enough radioactive decay that they could be removed from the water and taken to the chemical processing plant, to be chopped up, dissolved in acid and other solvents to permit extracting the plutonium. From there, the plutonium was turned into metal while the solvents – now highly radioactive – were stored in tanks, many of which are still awaiting final disposition.
The first goal was to extract enough plutonium to learn how to do this processing, then to make enough plutonium metal to learn its properties – how to mold it, shape it, how to work safely with it, and so forth. One early lesson was that it catches fire spontaneously – this meant that any machining or other work needed to be performed in an inert atmosphere (i.e. an atmosphere without oxygen). Another lesson was that it’s fairly toxic (https://www.ntanet.net/mythbusting-plutonium-facts-vs-fiction-revealed). And, then, when there was more plutonium, they started to do physics experiments to learn how much was needed to make a critical mass, and how to assemble a critical mass reliably.
Interestingly, while the reactor achieved criticality just as expected, after operating for a short time the power started dropping and it shut itself down! Fermi was apparently unsurprised – he’d suspected that some of the fission products might have neutron-absorbing properties. Eventually, they realized that one fission product in particular, Xe-135, was the culprit and they also learned how to deal with it – increasing the amount of uranium in the core to boost the number of neutrons so that they could “burn up” some to the xenon while still having enough to keep the reactor running (interestingly, a similar process contributed to the Chernobyl reactor accident 40+ years later). In any event, not only did they manage to do all of this – build the reactor, invent and build the chemical processing, learn about plutonium’s properties, design and make the weapon), but they did it all in less than three years…faster than we can write a contract today.
There’s a ton more I could write about the Hanford reactors, the chemical processing “canyons,” the Manhattan Project – as well as about the environmental pollution (chemical as well as radiological) – but this seems a good place to stop for today. But if you’re still interested, drop me another line and I can pick up another of the many threads of this story. For now, the bottom line is that the Hanford B Reactor was a shot in the dark, a genuinely daring venture that could well have come to nothing – but that worked amazingly well, considering how very little we knew at the time. And it held up amazingly well, producing plutonium through much of the Cold War. Considering the role it played in the development of nuclear weapons, as well as the environmental legacy left behind by other parts of the Manhattan Project, its historic designation is well-earned and well-deserved.